How GPS brings time to the world
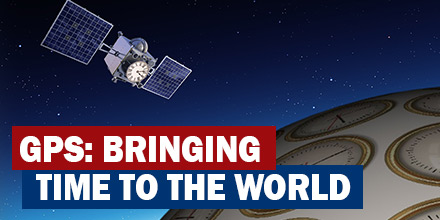
Knowing the correct time is something we take for granted. But, who exactly decides the correct time in the first place? How does anyone go about determining the correct time? And, how does GPS fit in to the story?
Ultimately, the International Bureau of Weights and Measures (BIPM, Paris) determines the correct time.
To determine the time, BIPM in Paris relies on contributions from a worldwide collaboration of timing laboratories. Each of these laboratories maintain their own measure of time and compare it with GPS time.
One clock to rule them all
Timing labs employ precise clocks. To measure time precisely, Cesium atomic clocks and Hydrogen masers are among the most popular devices.
Although these clocks are very reliable -- accurate to about 2 nanoseconds per day -- small variations still occur. At BIPM in Paris, they compare the performance of clocks in timing labs from around the world. They use a weighted average of all contributions and calculate Coordinated Universal Time (UTC).
Interestingly, labs with better performing or more stable clocks receive more weight in the UTC calculation.
This means that real-time UTC is only an approximation... albeit a very accurate one. Thus, they determine the more precise calculation in retrospect.
The Circular-T journal, published monthly by BIPM, contains the small corrections. They apply these corrections to UTC for the previous month.
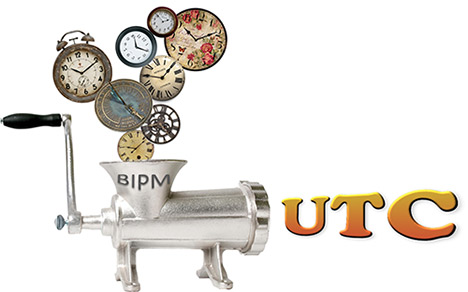
GPS receivers and time
Each timing lab contributing to UTC measures its own version of UTC. For example, UTCBrussels is the Belgian measure of UTC.
So how does BIPM compare the performance of all these different clocks?
It uses GPS receivers. Or, more accurately, GNSS (Global Navigation Satellite System) receivers which - in addition to GPS -- track constellations, such as: GLONASS, Galileo, BeiDou and IRNSS.
The precise measurement of time is at the heart of every GPS receiver.
They determine the distances between satellite and receiver, used to calculate position, by measuring the transit times of the satellite signals to the receiver.
An error of 1 nanosecond in the transit time translates into an error of 30cm in the distance.
Flying clocks
The GPS satellite constellation uses its own precise measure of time called: GPS time. Each GPS satellite has its own, on-board set of atomic clocks. Thus, satellites are also very accurate flying clocks.
By tracking a GPS satellite, a receiver can record the time differences between its own receiver clock and the satellite clock, e.g. UTCBrussels - GPS time.
The time differences, along with other information, are in a data format called CGGTTS and sent to BIPM. Using CGGTTS and other data, BIPM compares a clock in Brussels with a clock in New York by subtracting the individual differences with GPS time. As such, this technique is known as "common view".
UTCBrussels - UTCNew York = (UTCBrussels - GPS time) - (UTCNew York - GPS time).
The two GPS time terms above cancel each other out leaving the difference between UTCBrussels and UTCNew York.
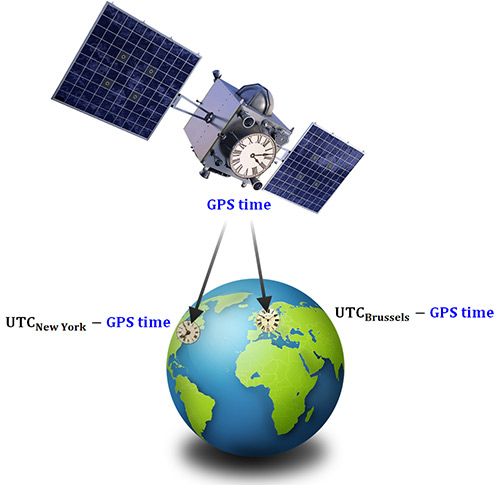
Setting up a timing laboratory
In order to compare the atomic clocks used in timing labs around the world, they need to connect to a GPS timing receiver. A GPS timing receiver uses an external atomic clock instead of its own clock; which it does by using two output signals from the atomic clock:
- a pulse every second synchronised to UTC (PPS IN) and
- a 10 MHz frequency reference that is essentially a sine wave (REF IN)
Figure 3 depicts the basic ingredients of a timing laboratory.
However, to reach the nanosecond accuracy required, it takes a great deal of expertise and preparation.
Signal delays in all elements in the setup require accurate calibration. To do this, BIPM maintains a set of pre-calibrated travelling receivers as calibration references.
As well as providing 1/3 of the timing receivers used for the calculation of UTC, Septentrio also provides BIPM with timing receivers for calibration.
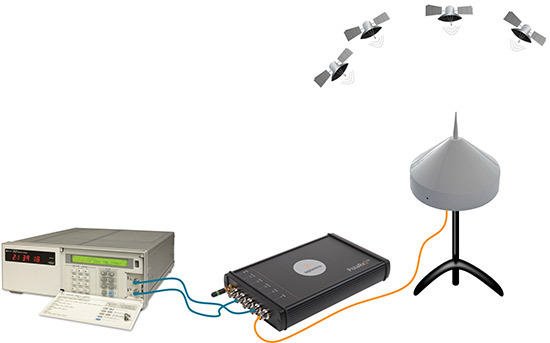
Pushing the boundaries of science
Beyond defining and disseminating UTC, GPS timing receivers are staking their place at the forefront of science.
For example, take the case of the T2K experiment. By precisely measuring the transit time of neutrinos between two locations, limits are placed on their mass. Thus, it sheds more light on the nature of these elusive particles.
At the other end of the size spectrum, the Very-Long-Baseline Interferometry (VLBI) technique uses radio telescopes at distant locations. These telescopes are linked together in networks by time-synching their observations using GPS common view. The resulting resolution is far in excess of anything that can be achieved by any single telescope on its own.
GPS technology continues to find new ways to improve our world and advance our knowledge of it.